Author: Sissel Martinsen
Whether you receive an EOS or you yourself have developed an EOS, it is always a good idea to quality check the EOS. You want to make sure that the EOS calculates physically consistent properties and thermodynamic valid solutions, and that it does not give any non-physical three-phase solutions that will slow down your simulation.
Consistent Physical Properties:
Plots to study physical consistency:
- Liquid density at standard conditions versus molecular weight (Mi) or normal boiling point (Tbi) for C6+ components
- Liquid viscosity versus Mi or Tbi for C6+ components
- Tbi versus carbon number or molecular weight for C6+ components
- Critical temperature and pressure versus Tbi or Mi
When tuning an EOS, critical properties (Tci, pci, ωi) of the C7+ components may be adjusted to improve phase behavior predictions of lab PVT data. If the corresponding volume shifts si are held constant – and not re-fit to component specific gravities – then the (Tci, pci, ωi) changes can result in non-physical liquid densities of C7+ fractions calculated from the EOS model. You might end up with surface oil densities decreasing instead of increasing during depletion of an oil reservoir. Or even worse, predicting reservoir gas densities that are higher than reservoir oil densities! This can happen if you regress on volume shift factors of pure components (C1, C2, C3, …) and match reservoir oil densities while forgetting to match the reservoir gas densities. As a rule of thumb, component properties should not be changed for pure, defined components, including aromatic BTEX components like Benzene and Toluene.
A plot should be made of EOS-calculated liquid density at standard conditions (or specific gravity, gi) for C6+ single carbon number (SCN) components versus molecular weight. This SCN relationship is expected to monotonically increase; non-monotonicity will be seen for pure-compound isomers in the C6-C9 range (when used in an EOS model). If SCN true boiling point distillation data is available and shows slight non-monotonic density versus molecular weight trend, this trend can be honored by the EOS. When such data are not available, a monotonic gi –Mi trend is expected for SCN components.
In a previous monthly article (LBC Viscosity Correlation for Gas Condensate Reservoirs) from whitson, we mentioned the importance of tuning critical volumes to create a physical consistent viscosity model using the LBC correlations. The recommended in the article were:
- For each & every C6+ fraction (Cx), run your PVT software using a feed that is set to 100% Cx, calculating the oil viscosity at reservoir (relevant) temperature and one atmosphere. Two physical constraints are considered: (a) the viscosities of each fraction monotonically increase, or very nearly so, and (b) the heaviest fraction should always have the highest viscosity.
- Plot the fourth-degree polynomial (right hand side) of the LBC reduced density correlation using the five LBC constants (original or tuned). The function should be (a) monotonically increasing with reduced density, and (b) not deviate significantly from the original LBC correlation at lower reduced densities (𝜌r<0.5) describing gaseous fluids.
- Simulate a constant composition expansion and make sure that saturated oil viscosities are always higher than saturated gas viscosities at the same pressure.
![]() | ![]() |
The EOS giving the calculated densities and viscosities presented in Fig. 1 used adjustments of critical volume in the viscosity tuning, but only for the C7+ components. C6 has a higher viscosity than C7 which is likely not physical but results because the C6 and C7 were treated inconsistently in the LBC tuning process.
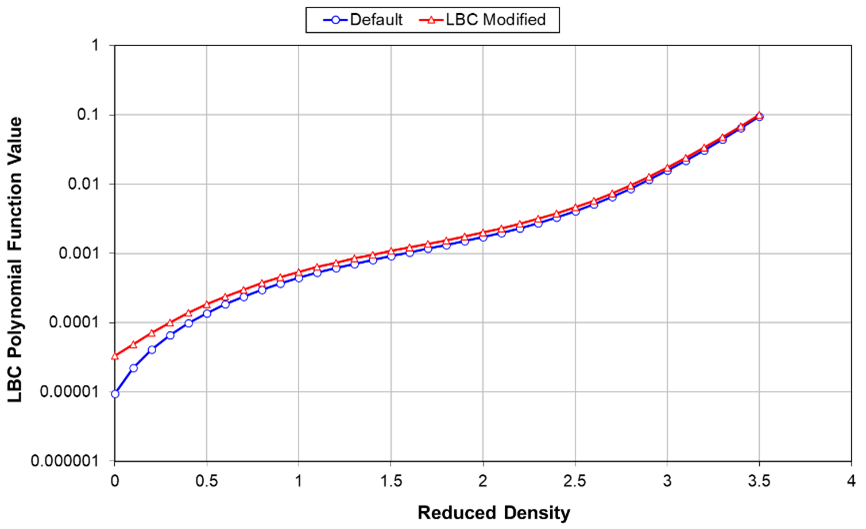
Above, the figure shows that LBC constants were tuned to match measured viscosities, and the LBC polynomial function is monotonic with reduced density, implying consistent viscosities. However, the largest change in the LBC relationship is for the lightest components controlling mainly gas viscosities. We normally recommend adjusting only the LBC coefficients for the highest-order terms, leading to a larger impact on oil viscosities and retaining the expected accuracy of the original LBC correlation for gaseous fluids.
Normal boiling points for single carbon numbers and lumped components should monotonically increase with increasing molecular weights. Aromatics will have higher normal boiling points versus molecular weights compared with lumped components consisting of a mixture of paraffins, naphtenes, and aromatics.
Monotonic critical temperature and critical pressure versus normal boiling point behavior is common, but not a necessity. An EOS with critical pressure monotonically decreasing with increasing boiling point can give a thermodynamic inconsistent model. Likewise, a non-monotonic critical pressure versus boiling point trend can give a thermodynamic consistent EOS model.
Thermodynamic consistent EOS model
A thermodynamic consistent EOS model is a model that gives consistent phase behavior. This normally is guaranteed by not-crossing K-values amongst hydrocarbons. Crossing K-values would suggest that neighboring components change their relative preference to partition into the gas and oil phases as a function of pressure. Although such crossing-K-value behavior can exist for isomers of a given carbon number, they seldom are found for SCNs.
We suggest making a plot of K-values at saturation pressures and separator pressures versus either (a) normal boiling points or (b) K-values from a flash at surface conditions. The plot should yield monotonic trends for single carbon numbers and lumped components at all pressures. Non-monotonicity between isomers and paraffins is not uncommon. CO2and H2Smay also show non-monotonicity without this indicating inconsistency. However, if a non-monotonic trend between components from the same family (e.g. paraffins only or lumped single carbon numbers) is observed, this is a potential red flag of inconsistency.
Plotting the K-values versus pressure (from a constant composition expansion experiment) for all the components in the EOS is recommended. K-values should not “cross” in the pressure range from 1 atm to saturation pressure. If crossing K-values are observed, the binary interaction parameters (BIPs) are often the cause. BIPs have a minor role on the calculated K-values at pressures below 1000 psi. However, they play a key role at higher pressures. Non-monotonic BIPs, and particularly neighboring BIPs with opposite signs, may lead to non-physical K-value behavior.
It should be noted that even zero binary interaction parameters, or smooth, consistent BIPs can have minor K-values crossing for SCN isomers. Fig. 3 shows crossing K-values from an SRK EOS with zero BIPs and only pure components with unregressed library component values.
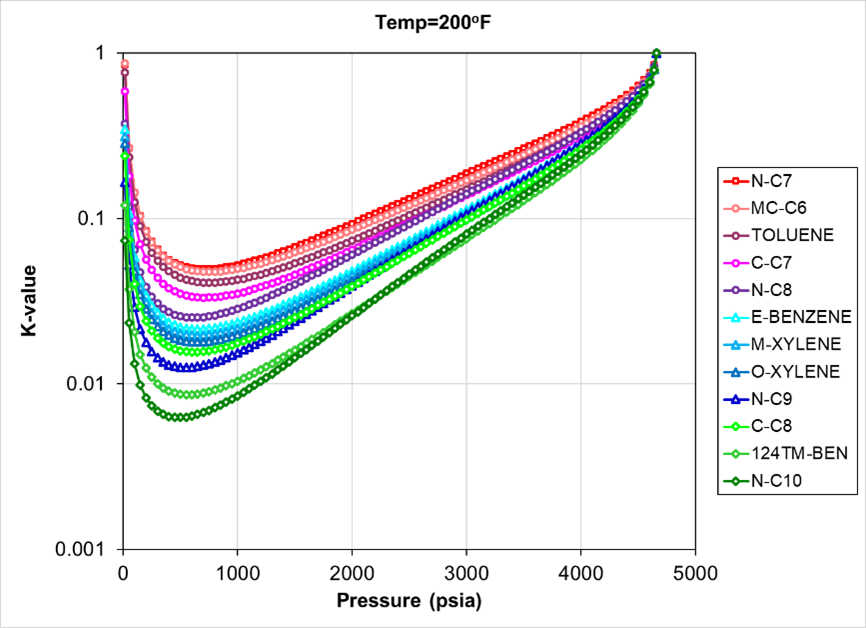
Fig. 4 shows EOS calculated K-values from a CCE experiment using a single carbon number SRK EOS with no BIPs. This EOS gives, as expected, no crossing K-values, since a similar paraffinicity factor is assumed for all C7+ components.
![]() | ![]() |
References
Younus, B., Whitson, C. H., Alavian, A. et al. 2019. “Field-wide Equation of State Model Development.” Presented at the URTeC, Denver, Colorado, USA, URTeC: 551.
Martinsen, S. Ø., Castiblanco, I., Osorio, R. et al. 2010. “Advanced Fluid Characterization of Pauto Complex, Colombia.” Presented at the SPE Annual Technical Conference and Exhibition, Florence, Italy, SPE 135085.
Learn more about our consulting capabilities
###
Global
Curtis Hays Whitson
curtishays@whitson.com
Asia-Pacific
Kameshwar Singh
singh@whitson.com
Middle East
Ahmad Alavian
alavian@whitson.com
Americas
Mathias Lia Carlsen
carlsen@whitson.com
About whitson
whitson supports energy companies, oil services companies, investors and government organizations with expertise and expansive analysis within PVT, gas condensate reservoirs and gas-based EOR. Our coverage ranges from R&D based industry studies to detailed due diligence, transaction or court case projects. We help our clients find the best possible answers to complex questions and assist them in the successful decision-making on technical challenges. We do this through a continuous, transparent dialog with our clients – before, during and after our engagement. The company was founded by Dr. Curtis Hays Whitson in 1988 and is a Norwegian corporation located in Trondheim, Norway, with local presence in USA, Middle East, India and Indonesia